https://www.shoulderdoc.co.uk/article/384
Rotator Cuff Biomechanics
Lennard Funk
For MSc Orthopaedic Engineering, 2005
For MSc Orthopaedic Engineering, 2005
The aim of this presentation is to discuss the current literature and opinions on the biomechanics of the rotator cuff and relate this to the clinical relevance in rotator cuff tears. I intend to cover:
- Cuff Mechanics
- Tendon Anatomy - layers, microanatomy, blood supply
- Pathomechanics of cuff tears
Rotator cuff Muscles
The shoulder complex comprises 30 muscles. These muscles both move the shoulder and stabilise it - 'movers' and 'shakers'. The rotator cuff muscles predominantly stabilise the glenohumeral joint, but also contribute significantly to movement.
The rotator cuff muscles are:
- Supraspinatus
- Infraspinatus
- Teres Minor
- Subscapularis
The tendons of these muscle coalesce to form the rotator cuff. The muscles are inseparable at this level, except for subscapularis which is separate and joined to the rest of the cuff via the rotator interval.

Supraspinatus
Supraspinatus is not only an initiator of abduction, but acts throughout the range of abduction of the shoulder. It has equal abduction power as deltoid. Note that it lies in the scapular plane - i.e. 30 degrees to the coronal plane (Figure 2).
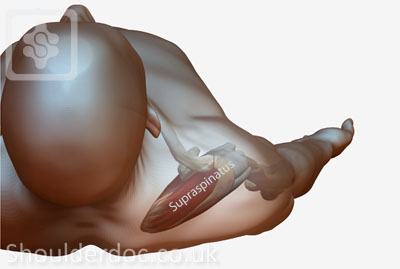
Infraspinatus & Teres Minor
These two muscles lies below the scapular spine and are external rotators of the shoulder. Infraspinatus primarily acts with the arm in neutral and Teres Minor is more active with external rotation in 90 degrees of abduction.
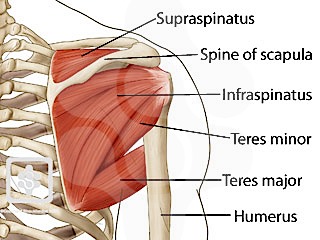
Subscapularis
Subscapularis is the main internal rotator of the shoulder. It is the largest & strongest cuff muscle, providing 53% of total cuff strength. The upper 60% of the insertion is tendonous and the lower 40% muscle. It is a passive restraint in neutral, but not abduction.
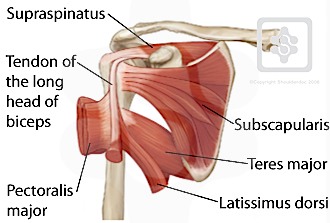
Deltoid
The deltoid muscle is the only shoulder elevator if the supraspinatus is torn and dysfunctional. Therefore most rehabilitation is directed towards this muscle. It comprises anteriormiddle and posterior portions which are more active depending on the direction of arm elevation.
Anterior view of deltoid Posterior view of deltoid
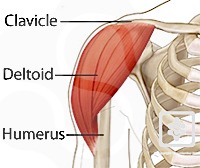
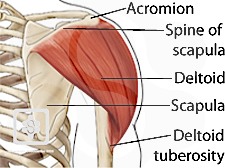
Free Body Diagram for calculating Deltoid Force
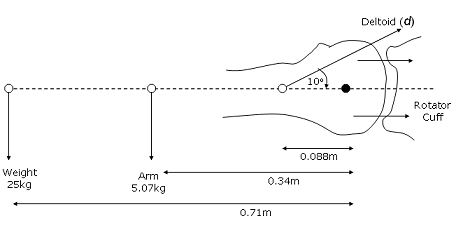
In this diagram, with the arm fully extended the deltoid has to counteract the weight of the arm and a 25kg weight in the person's hand.
1. Moments pulling humerus down:
(25 x 9.81) x 0.71 = 174Nm
(5.07 x 9.81) x 0.34 = 16.91Nm
= 174 + 16.91 = 190.91Nm
2. Moments puling humerus up:
Deltoid(d) x sin10o x 0.088 = 0.01528d Nm
Assume equilibrium: moments up = moments down
190.91 = 0.01528d
Deltoid tension d = 12 494.11N
Now if the person bends his elbow, reducing the moment arm of the 25kg weight and arm the force required of deltoid to elevate the arm will be reduced.
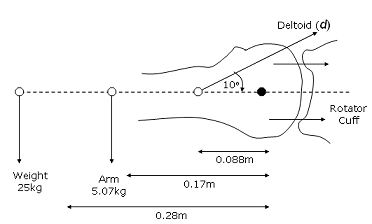
(25 x 9.81) x 0.28 = 68.67Nm
(5.07 x 9.81) x 0.17 = 8.46Nm
= 68.67 + 8.46 = 77.13Nm
2. Moments puling humerus up:
Deltoid(d) x sin10o x 0.088 = 0.01528d Nm
Assume equilibrium: moments up = moments down
68.67 = 0.01528d
Deltoid tension d = 4 494.1N
Force Couples
A force acting on a body has two effects, one to move it and two to rotate it. However, a body may rotate without moving. I.e. a force can cause only rotation with out translation. A force couple is a system that exerts a resultant movement, but no resultant force. Two equal and opposite forces exert a purely rotation force. In the shoulder the body is the humeral head and the equal but opposite forces are the rotator cuff muscles.
In a force couple, force generated by one muscle (the primary agonist) requires the activation of an antagonistic muscle so that a dislocating force does not result (Nordin & Frankel, 2001).
The rotator cuff muscles act as a force couple with each other and the deltoid. The rotator cuff muscles work together to contain the glenohumeral joint, which is an inherently unstable joint. The progression of a rotator cuff tear or dysfunction leads to superiorsubluxation of the humeral head. This leads to dysfunction of the shoulder.
The rotator cuff stabilises the glenohumeral joint through force couples in both the coronal and transverse planes.
Coronal Force Couple
![]() | ![]() |
Deltoid and supraspinatus both contribute to abduction equally. As the arm is abducted the resultant joint reaction force is directed towards the Glenoid. This 'compresses' the humeral head against the Glenoid and improves the stability of the joint when the arm is abducted and overhead. [ Parsons et al. J Orthop Res. 2002 ] |
Transverse Plane Force Couple
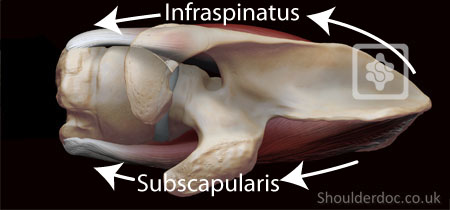
Throughout the range of motion the compressive resultant joint reaction force in the transverse plane contributes to joint stability. This is the predominant mechanism resisting superiorhumeral head displacement with cuff tears. As long as the force couple between subscapularis and Infraspinatus remains balanced the joint remains centred. [Parsons et al. J Orthop Res. 2002]
Static Restraints
In addition to the dynamic stabilisers mentioned above, there are important secondary restraints to superior displacement of the humeral head with cuff tears.
Coraco-acromial Arch
The coraco-acromial arch is the combination of the coracoid, coracoacromial ligament and acromion. These form an arch above the rotator cuff and humeral head.
![]() | Coraco-acromial arch (green) formed by the coracoid, coracoacromial ligament and acromion |
Long Head of Biceps
The long head of biceps passes over the humeral head curving in two planes forming the shape of a question mark. It is recognised as providing a small degree of stability to the gleno-humeral joint. This is predominantly with abduction and external rotation of the arm in the scapular plane [ Warner & McMahon, JBJS, 1995 ; Itoi et al. JBJSB, 1993 ].
The biceps pulley is a stabiliser of the long head of biceps in the biceps groove. Rupture of this pulley with a rotator cuff tear leads to medial subluxation of the long head of biceps and dysfunction.
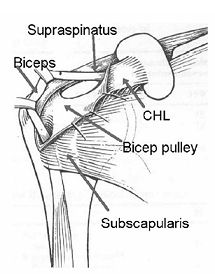
Rotator cuff patho-anatomy
Cuff Ultrastructure
The fusing of the rotatorcuff tendons suggests that they act more as a combined and integrative structure than as single entities. The microstructure of the rotator cuff tendons near the insertions of the supraspinatus and infraspinatus has been further described as a five-layer structure:
- Layer one is composed of the superficial fibers of the coracohumeralligament.
- Layer two, which is the main portion of the cuff tendons, is seen as closely packed parallel tendon fibers grouped in large bundles extending directly from the muscle bellies to the insertion on the humerus.
- Layer three is also a thick tendinous structure but with smaller fascials than in layer two and a less uniform orientation.
- Layer four is composed of loose connective tissues with thick bands of collagen fibers running perpendicular to the primary fiber orientation of the cuff tendons. This layer contains the deep extension of the coracohumeral ligament and has been variously described as a transverse band, a pericapsular band, or a rotator cable. This layer may have a role in the distribution of forces between tendinous insertions and may explain why some rotator cuff tears are clinically asymptomatic.
- Layer five is the true capsular layer and forms a continuous cylinder from glenoid to humerus. The fibers in this layer are, for the most part, randomly oriented.
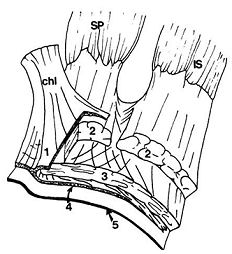
The fibre orientation also differs along the length of the rotator cuff tendon. Near the musculotendinous junctions, the tendons are composed mainly of parallel homogeneous collagen fibers but become flat ribbonlike bundles of fibers that cross at an angle of about 45 degrees as they reach insertion into the humerus [Gohlke et al. ]. Because of the various fiber orientations and distinct layers within the superiorcapsular complex, significant shear forces likely exist and may have a role in cuff tears. These intratendinous variations in the cuff structure may explain why intrasubstance tears occur. Shear forces are probably directed to layer four, which is the site of development of intratendonous cuff tears. These tend to be degenerate tears of the cuff.
Collagen
The midsubstance of the supraspinatustendon is primarily composed of Type I collagen, with relatively small amounts of Type III collagen, decorin, and biglycan. The fibrocartilage portion of the insertion has a collagen and proteoglycan content similar to that of tissues that have been subjected to compressive loads. This is partly due to the wrapping of thetendon around the humerus. Therefore, it mainly contains Type II collagen and larger proteoglycans such as aggrecan. The histological organization, however, does not resemble mature fibrocartilage. In rotator cuff tendinopathy, an increase in collagen Type III, a protein that plays a role in healing and repair, and glycosaminoglycan and proteoglycan content has been observed. These compositional changes may be adaptive, pathologic, or both, and are found to be altered in the older population.
Furthermore, recent studies have shown increased levels of smooth muscle actin (SMA) in torn rotator cuffs. SMA-positive cells have been shown to contract a collagen-glycosaminoglycan analog in vitro. SMA-containing cells in rotator cuff tears may react with the high levels of GAG and proteoglycan resulting in retraction of the ruptured rotator cuff and inhibition of potential healing.
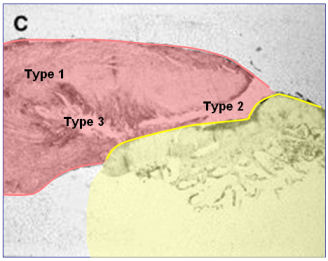
Vascularity
The major arterial supply to the rotator cuff is derived from the ascending branch of the anterior humeral circumflex artery, the acromial branch of the thoracoacromial artery, as well as the suprascapular and posterior humeral circumflex arteries.
The pathogenesis of rotator cuff tears has been considered to be influenced by the microvascular supply of the rotator cuff tendons. Most cadaver studies have demonstrated a hypovascular area within the critical zone of the supraspinatustendon. It has been suggested that this area of hypovascularity has a significant role in the attritional degeneration of the aging tendon. More recent studies of the microvascular supply to the supraspinatus tendon in symptomatic patients with impingement syndrome suggest that in the area of greatest impingement, i.e., the critical zone (8mm proximal to the insertion of the supraspinatus tendon), there is actually hypervascularity. In contrast to the cadaver investigations, these studies seem to imply that hypervascularity or neovascularization is associated with symptomatic rotator cuff disease secondary to mechanical impingement[ Chansky & Iannotti, Clin Sports Med. 1991 ].
In vivo analysis using orthagonal polarisation spectral imaging has demonstrated that there is good vascularity of supraspinatus, even in the critical zone in intact rotator cuffs [Biberthaler et al. JBJSA. 2003 ].
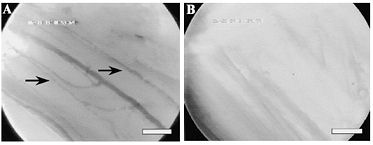
A - capillaries within normal supraspinatus tendon. B - absent capillaries in the edges of a supraspinatus cuff tear.
Aetiology of cuff tears
There are two main theories for the cause of rotator cuff tears:
- Extrinsic - due to compression and impingement of the rotator cuff from without. Such as on the subacromial bursal side from acromial spurs and the coracoacromialligament (subacromial impingement); and on the articular side from trapping of the tendonbetween the Glenoid and humerus in extreme abduction and external rotation (internal impingement)
- Intrinsic - development of tears due to changing properties of the rotator cuff itself.
We will explore some of the biomechanical reasons for the development of cuff tears, rather than the differences between extrinsic and intrinsic causes.
Strain
Increasing strain in supraspinatus up to 60 degrees abduction using MRI.
No difference in strain between bursal & articular side of the tendon [Mehta et al. JBJS. 2003 ].
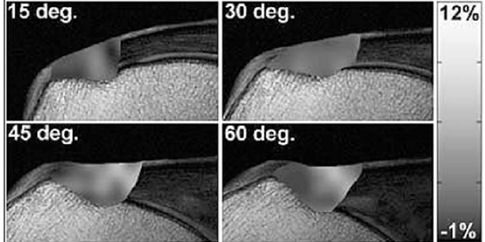
Stress Concentration
Using finite element modelling of the rotator cuff the stress concentrations were studies in varying degrees of subacromial impingement. The stress concentrations were highest in the critical zone of the cuff with tears potentiating on the articular side, bursal side and intratendinous. Articular side tears were slightly more common [Mehta et al. JBJS. 2003 ].
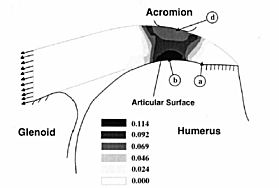
Subacromial Extrinsic Impingement
Neer originally believed that rotator cuff tears arose fom a mechanical process secondary to progressive wear. He found theanterior aspect of the acromion involved with or without osteophytes from the AC joint [ Neer, CORR, 1982 ]
The morphology of the anterior acromion has been found to correlate with cuff tears. A cadaveric study of 140 shoulders that 73% of rotator cuff tears found were in type 3 hooked acromions [Bigliani, Clin Sports Med, 1991 ]. This has also been borne out by recent clinical studies where acromial morphology was found to be a predictor for cuff tears [ Gill et al. JSES, 2002 ].
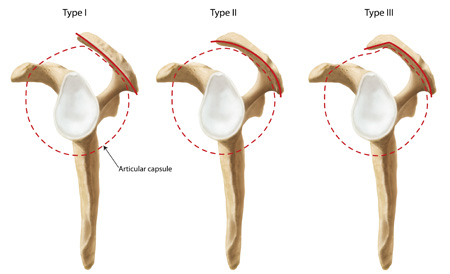
Bigliani classification of acromial morphology. Type 3 was more commonly associated with rotator cuff tears [Bigliani, Clin Sports Med, 1991 ]
Internal Impingement
The triad of anterior capsular laxity, posterior contraction and internal impingement was originally described in overhead athletes [ Walch et al. 1991 ; Jobe 1995 ]. The internal impingement occurs when the cuff is pinched between the humeral head and the postero-superiorlabrum during extreme abduction and external rotation. This scuffs and abrades the articular surface of the cuff progressively leading to cuff tears.
Tendon Degeneration
The rotator cuff appears to degenerate with age. On magnetic resonance (MRI) studies cuff tears were present in 54% of asymptomatic people over the age of 60 years [ Sher et al. 1995 ]. However, MRI is only 75-90% accurate in diagnosing full thickness rotator cuff tears [ Shroder et al. 2003 ;Teefey et al. 2004 ] . More work needs to be done on the age-related changes of the rotator cuff, but the aging cuff is probably more prone to the extrinsic and intrinsic mechanisms.
Summary
The aetiological and pathomechanical factors in the development of rotator cuff tears appear to have multifactorial roots. An understanding of the anatomy, mechanics and the biology of the rotator cuff is essential to our treatment plans.
Комментариев нет:
Отправить комментарий